As the commercial HVAC industry shifts toward decarbonization and increased sustainability, the role of mechanical design engineers is evolving rapidly. For new mechanical engineers entering the field, the amount of information to learn can be overwhelming. One critical decision they must acknowledge is how to serve a building's heating loads. This article focuses specifically on hydronic system designs for buildings, providing a high-level comparison of fossil-fuel-fired boilers, electric boilers, and various types of heat pump technologies. Key factors are discussed, such as heating water system temperatures, local regulations, and owner requirements, sustainability goals, availability and cost of site utilities, heat recovery capability, and building location. This is to inform the decision-making road map mechanical engineers create and offer to the building owners as viable options.
The first key factor that readers will want to understand when comparing hydronic heating equipment, is coefficient of performance (COP). The COP equation is shown below. Higher COPs corresponds to increased operating efficiencies.
COPs for the hydronic heating equipment discussed in this article are listed below. Even though the COPs of heat pump-type equipment are significantly higher, it is vitally important to understanding the tradeoffs of using heat pumps in lieu of or in combination with boilers.
Fossil Fuel-Fired Boilers
Fossil-fuel-fired boilers are the more conventional approach to building heating, and their design has improved over the years. The minimum boiler efficiency by ASHRAE 90.1 (2010) ranges from 79% to 84% (efficiency as of March 2, 2020 per table 6.8.1F).
Fossil-fuel-fired boilers come in an array of sizes and configurations, including condensing and noncondensing boilers. Condensing boilers can achieve over 90% efficiency when designed with the appropriate water temperature, whereas non-condensing boilers can only achieve about 70% efficiency. Condensing boilers capture the heat of vaporization by condensing wasted water vapor and repurpose that amount of heat for water heating, thus achieving higher efficiency. Condensing boilers have a higher initial cost due to the added corrosion resistance and treatment requirements for the condensed water but can generally pay back over time through lower operational costs (data based on a lifecycle cost analysis). The operating temperature for typical gas-fired boilers is between 42◦ to 195◦F. For condensing boilers, the lower the temperature, the more the boiler can take advantage of the condensing capability and higher efficiency.
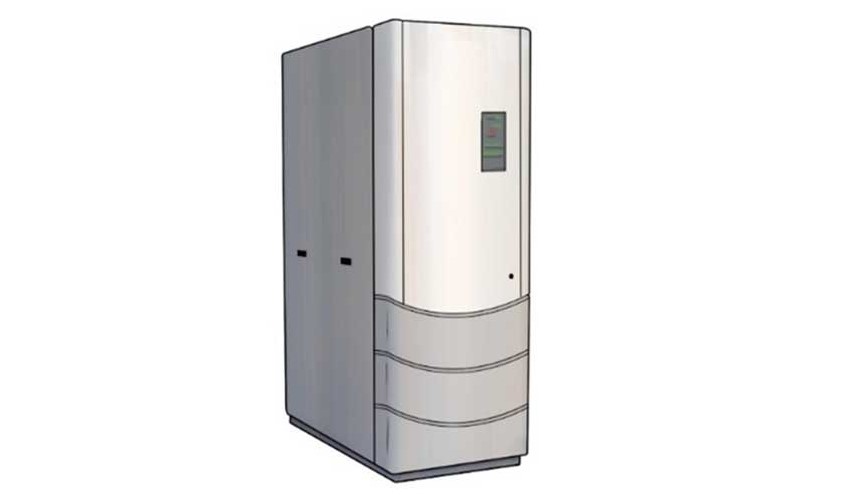
Fossil-fuel-fired boilers can also be categorized by fuel types, typically using propane or natural gas. There are also dual-fuel boilers that are designed to serve as a backup fuel source during a utility outage. Building facilities that require site resilience, such as hospitals or data centers, will typically employ dual-fuel boilers in consideration of continuous operation.
Boiler designs must comply with applicable regulatory codes and regulations. Commonly, boiler construction must meet the ASME Boiler and Pressure Vessel Code. Safety valves are required for fossil-fuel-fired boilers to provide overpressure protection. Each valve must be listed and labeled with minimum capacity associated with the equipment served. They also should be set at a maximum pressure not surpassing the equipment nameplate pressure. Code requirements specify boiler outdoor combustion air locations as well as overall dimensions. Since gas-fired boilers are devices that burn fossil fuels and carbon monoxide is an inherent byproduct of combustion, there are also regulations pertaining to carbon monoxide exposure. For example, if the boilers are in a residential, hotel, or hospital patient wing, a carbon monoxide alarm should be installed within 10 feet of each room for sleeping purposes.
Special code provisions may govern boiler room designs for specific facility types. For example, Florida’s State Requirement for Educational Facilities stipulates the boiler room must be separated from the rest of the building a one-hour fire wall. The boiler room is required to be connected to the building exterior by an out-swinging door. The boiler room also cannot be connected to any student corridor.
Fossil-fuel-fired boilers require flue vent pipes, which will limit the physical location options for the boilers and create coordination challenges. A double-wall flue vent pipe is required to reduce the surface temperature and alternate materials of construction for corrosion resistance. The National Fuel Gas Code (NFGC), ANSI Z233.1, NFPA 54, and manufacturers’ installation manuals will typically provide guidance regarding vent pipe design criteria.
When it comes to boiler controls and operational safety, designers typically reference two key standards from ASME and NFPA. ASME CSD-1, Controls, and Safety Devices for Automatically Fired Boilers, outlines requirements for smaller boilers with a fuel input rating of 12.5 million Btu per hour or less. The control and safety devices associated with larger boilers — those with a fuel rating equal to or greater than 12.5 million Btuh — are regulated by NFPA 85, Boiler and Combustion Systems Hazards Code. The control and safety devices for smaller boilers (fuel input rating of 12,500,000 Btu/hr or less) shall meet the requirements of ASME CSD-1, Controls, and Safety Devices for Automatically Fired Boilers. The control and safety devices for larger boilers (fuel input rating equal to or greater than 12,500,000 Btuh) shall meet the requirements outlined by NFPA 85, Boiler and Combustion Systems Hazards Code.
Electric Boilers
Electric boilers have had an increased market share in the past few years. One driver is a response to government regulations and incentives related to carbon footprint and greenhouse gas emissions (GHG). Unlike fossil-fuel-fired boilers, electric boilers do not have burners, and they rely on electric resistance to increase water temperature.
Both steam and heating hot water electric boilers are available in the current market. Electrical boilers typically have 95% or above efficiency. Since electrical boilers do not have combustion products, no stacks (flue vent pipes) are required for installation. This could not only provide a cost-saving advantage but also provide space planning flexibility. The operating temperature for typical electric boilers is 45◦ to 245◦.
Electrical boilers differ in physical footprint than fossil-fuel-fired boilers. Electrical disconnect size and clearance also need to be accounted for during mechanical space planning.
The transition to electric boilers may be a response to regulatory shifts. For example, New York City has banned gas connections to new buildings in 2021. In other states, incentives are put in place to replace fossil-fuel-fired systems in existing buildings. The main barrier of adopting electric boilers is the cost implication. Natural gas is typically a fraction of the cost per energy unit compared to electricity. Another concern is the increase of demand on the electrical grid. According to McKinsey, electricity demand may triple by 2050, which will surpass the maximum capacity of existing infrastructures.
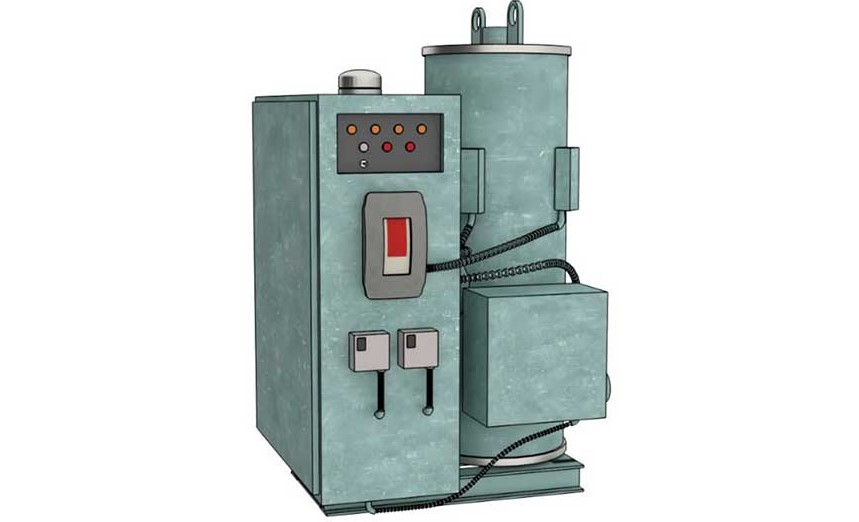
Packaged electric boilers are required to be listed and labeled in accordance with UL 834, UL Standard for Safety Heating, Water Supply, and Power Boilers – Electric. The standard covers electric boilers rated at 15,000 volts or less, which includes an array of applications.
Due to the rise of decarbonization and electrification design trends, popularity of electrical boilers has increased. The market shift in building electrification is impacting increased coordination between utility companies, building owners, and engineers. For instance, building electric peak load could shift from summer to winter as more buildings are electrified. This could result in changes in utility maintenance schedules and demand-based electricity rate structures.
Heat Pump Technologies
A heat pump is a vapor compression device that takes heat from the evaporator (source) and moves it to the condenser (sink) using refrigerant and compressors. There are multiple types and sub-types of heat pumps to choose from. There is also a lack of consistent language in the industry when discussing and describing the various types and functions of heat pumps. For this article, we’ll discuss water-source and air-source heat pumps as two larger categories of heat pumps and further discuss modes of operation available with each type. When engineers decide to include a heat pump in their hydronic design, they should complete extensive research on other heat pump characteristics, such as compressor types, modular capabilities, refrigerant options, minimum flow requirements, control strategy, and pumping configurations.
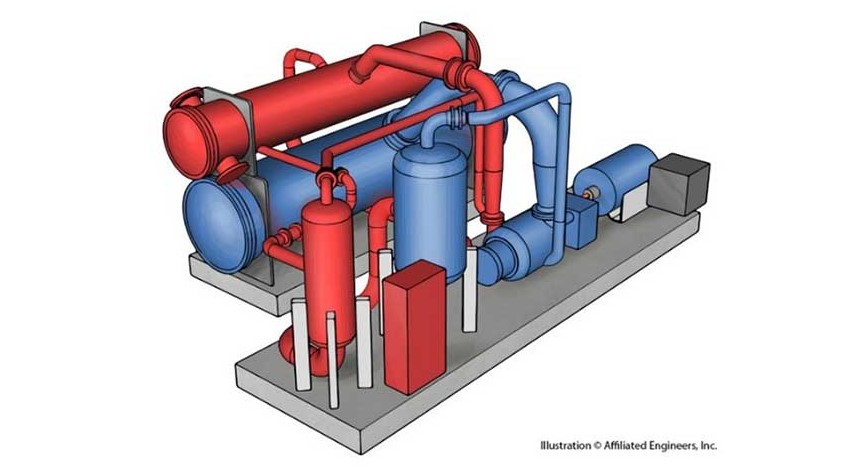
Heat pumps can operate in three modes of operation: heating only, cooling only, and simultaneous heating and cooling. The mode options available for a heat pump depend on the type of heat pump, such as air-source or water-source. Here's a breakdown of how different types of heat pumps operate in each mode:
- Heating only: In heating-only mode, the heat pump extracts heat from the heat sink (outside air or ground) and transfers it to the heating water system. The compressor pumps refrigerant through the condenser, where it absorbs heat energy. The refrigerant then moves through the heat pump’s evaporator, where it releases heat into the heating water system.
- Cooling only: In cooling-only mode, the heat pump absorbs heat from the chilled water system and rejects it to the condenser. The condenser can reject heat to different types of heat sinks (outside air, ground, domestic hot water, condenser water, etc.) depending on the type of heat pump that is provided.
- Simultaneous heating and cooling: Simultaneous heating and cooling mode is also known as heat recovery mode or dual-source mode. This mode allows the heat pump to simultaneously provide heating and cooling by optimizing the transfer of heat energy between the heating water and chilled water systems.
When designing around heat pumps with heat recovery capabilities, mechanical engineers must study the balance of heating and cooling loads within the building. Heat pumps need 20% to 30% minimum load on both sides (heating and cooling) to enable heat recovery. This study should also include the availability of waste heat sources (data centers, process equipment, technology equipment rooms, etc.) and heat rejection sources (terminal reheat coil serving zones with high ventilation requirements). Understanding the balance of simultaneous heating and cooling loads will ensure all captured waste heat is effectively utilized.
Air-source heat pumps (ASHP) are a type of heat pump that reject and/or absorb heat from the surrounding ambient air and must be located outdoors. ASHPs can operate in heating-only or cooling-only modes and can be specified to include heat recovery mode, making these types of heat pumps very flexible but also costly. A less costly air-source heat pump can be specified as a two-pipe ASHP where heat recovery mode is not included, but the heat pump can operate to produce either heating water or chilled water depending on its settings.
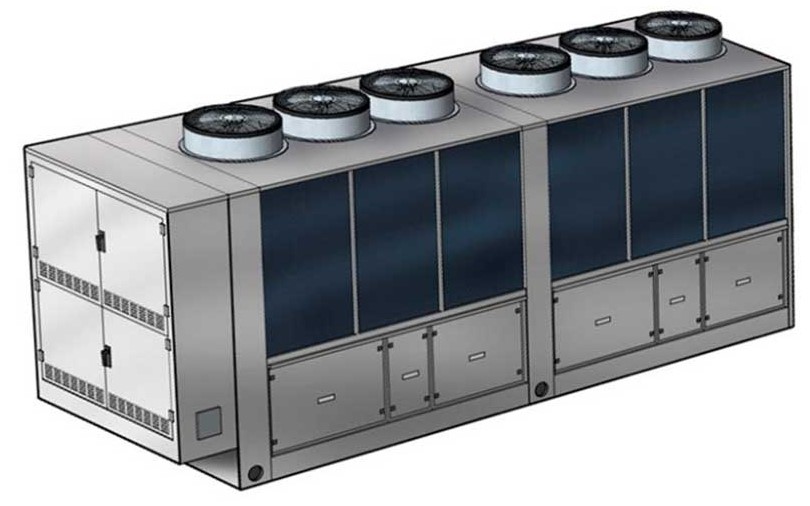
All ASHPs are subject to fluctuations in ambient air temperature, which can affect their performance. In general, at outside air temperatures less than 40◦, ASHPs will experience reduced heating capacity, lower operating efficiencies, and possibly lower heating water temperatures due to the decreased availability of heat in the air. To address this issue, manufacturers have developed advanced technologies, such as defrost cycles and supplemental electric heating, to improve ASHP performance in colder conditions. When designing around ASHPs, mechanical engineers must study how heating capacities and heating water temperatures are derated at colder outside air conditions. This often leads to oversized ASHPs and/or supplemental heating equipment (such as a boiler).
In contrast, water-source heat pumps (WSHP) are designed with heat sources and heat sinks that reject and/or absorb heat from hydronic systems. WSHPs are typically located indoors but can be specified with an enclosure that allows it to be located outdoors. A four-pipe WSHP utilizes two hydronic systems, typically the chilled water and heating water systems, as its heat source and sink. A six-pipe WSHP utilizes three hydronic systems to balance heating and cooling loads. Other hydronic systems that WSHPs could be connected to include domestic hot water systems, geothermal systems, condenser water systems, or other types of hydronic systems that are designed to capture and transfer heat energy.
Working in Combination
Often, the most suitable option for designing an efficient and cost-effective hydronic building heating system involves some combination of multiple system types. For example, for a healthcare clinic in the Pacific Northwest, a three-mode ASHP (cooling-only, heating-only, and heat recovery modes) with air-cooled chillers and a natural gas boiler was included in the initial design. The chillers and boiler were sized to handle peak heating and cooling loads beyond what the ASHP could handle during periods with simultaneous heating and cooling loads. After cost feedback, the design was modified to provide a two-mode (cooling-only and heat recovery) ASHP. It was acceptable to the design team to remove the heating-only mode of the ASHP, knowing that the natural gas boiler could serve all peak heating loads.
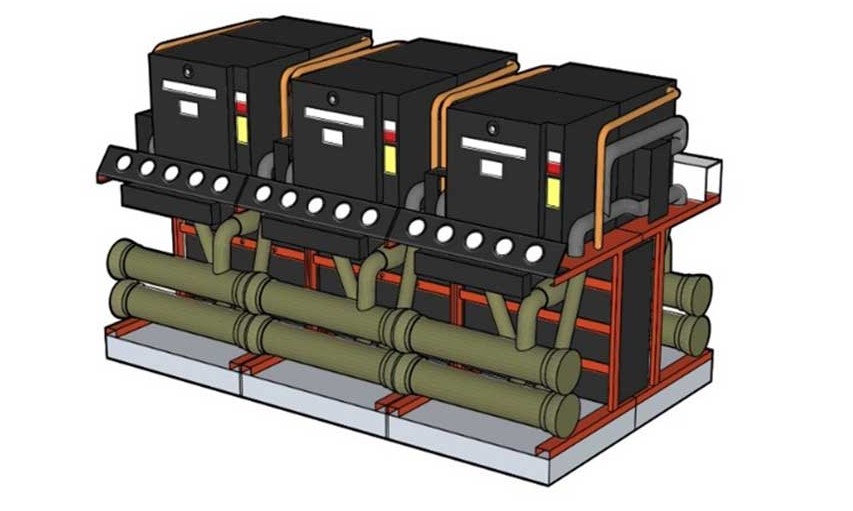
For a government laboratory building in the Pacific Northwest, the design included a six-pipe WSHP, connected a geothermal hydronic system, and a two-mode ASHP (heating-only and cooling-only modes). The control sequences state that the cooling-only mode of the ASHP provides trim cooling capacity to the building during peak cooling loads. In addition, the heating-only mode of the ASHP provides trim heating capacity during non-peak heat loads. The mechanical system is designed such that the heating-only mode of the ASHP only needs to operate at outside air temperatures that optimize its performance.
For a high-rise laboratory building in the Pacific Northwest, multiple four-pipe WSHPs, multiple two-pipe ASHPs, and an electric boiler are provided to meet local electrification code requirements. The four-pipe WSHPs are limited to one mode (heat recovery) but are connected to hydronic coils in the exhaust AHUs that allow the hydronic system to absorb or reject heat in the building’s exhaust airstreams depending on the valve position at these hydronic coils. The “two-pipe ASHPs provide additional heating and cooling capacity during peak loads. The electric boiler is only engaged during peak heating loads and when the ASHPs are cycling through their defrost cycles. To provide further design mitigation with the defrost cycles of the ASHPs, the hydronic system is also designed to operate with lower heating water temperatures (113◦ ) than what is typically provided in other systems.
Hydronic heating system design is also a balance between efficiency and resiliency. For a lab building that is located where sporadically low-temperature snow weather may occur, it was designed with a higher entering water temperature for a condensing boiler due to concerns with resiliency during low-temperature winters. To balance the resiliency with energy efficiency, the control sequence of the boiler integrated a temperature reset sequence so that higher entering water temperature will only be activated when outside air temperature is under a certain threshold.
Local climate can also dictate the building heating design options. For a marine science building located in the Southeast’s hurricane-prone region, an air-sourced heat pump is excluded as a viable option, due to hurricane and corrosion concerns for the outdoor equipment.
There are other building heating options beyond the ones discussed in this article, such as campus steam, VRF/heat pumps, zone-level electric resistance, etc. Zone-level electric resistance is preferred to electrical boilers in many cases, due to reduction of pumps and hydronic piping and insulation. However, if the heating system is required to be on emergency power, electric boilers are more stable than zone-level electric resistance.
With electrification goals or requirements becoming more prevalent, serving the building's heating loads present unique challenges for mechanical engineers. The design team must discuss whether a 100% electric building is feasible or whether a mostly electric building is more practical. This question is particularly important when studying a building's peak heating loads. If decarbonization is a goal for the project, one may consider 90% electric heating plus 10% fossil fuel, where the fossil-fuel boiler(s) serve the 10% peak of the load that’s normally present throughout the year. Fossil-fuel boilers may also be considered in an all-electric building if it’s only being used for redundancy and doesn’t operate under normal operating conditions.
References
- https://www.ashrae.org/file%20library/technical%20resources/ashrae%20journal/2022journaldocuments/jan2022_08-11_letters_january-2022.pdf
- https://www.gminsights.com/industry-analysis/industrial-electric-boiler-market#:~:text=What%20is%20the%20low%20voltage,of%20low%20emission%20heating%20systems.
- https://www.mckinsey.com/industries/electric-power-and-natural-gas/our-insights/building-decarbonization-how-electric-heat-pumps-could-help-reduce-emissions-today-and-going-forward
- https://www.deppmann.com/blog/monday-morning-minutes/top-10-things-you-should-know-about-commercial-electric-boilers-part-2/
- https://www.csemag.com/articles/getting-boiler-design-right/
- Haddadin, Jacob. "Introduction to boilers for the entry-level engineer: Entry-level consulting engineers should understand the definition and applications of a commercial hydronic boiler." Consulting-Specifying Engineer, vol. 56, no. 8, Sept. 2019, pp. 44+. Gale Academic OneFile, link.gale.com/apps/doc/A609500335/AONE?u=nysl_me_cu&sid=googleScholar&xid=84e9078b. Accessed 8 Dec. 2022.
- https://www.greenbiz.com/article/what-electric-boilers-tell-us-about-electrifying-everything
- https://www.pmengineer.com/articles/87948-back-to-basics-venting-for-gas-fired-boilers