In the last year, we’ve been inundated with information related to the design of pandemic-ready HVAC systems. Some of it has contributed positively to the design process, but much of it has deepened the morass of conflicting information engineers encounter as they navigate through a project.
Don’t despair. Tools exist to help you through this process. Last year, John Varley discussed lean design methodology as it relates to waste in the design process. Project teams using lean methods often use a decision-making technique well-suited for ferreting out the best solution to the pandemic puzzle called Choosing by Advantages (CBA). Dr. Paz Arroyo has studied and implemented CBA for different applications, including choosing HVAC systems for a net-zero energy building (NZEB). In this article, both will collaborate to tackle this contingent and relevant decision that many offices, schools, and public buildings will need to make.
Choosing by Advantages
Developed by Jim Suhr, CBA is a decision-making system that includes definitions, principles, models, and methods for making sound decisions. CBA emphasizes the importance of basing decisions on the differences among advantages of alternatives. Proper use of CBA methods will lead to sound decision-making. As such, reading Suhr’s book is highly recommended. It will provide engineers with the correct framework for applying CBA. Further, it also offers a strong foundation to communicate the process to the other stakeholders, who may not be familiar with the process since it is not commonly used by design professionals. This paper is intended to introduce the concept and convince the reader of its efficacy in sorting through complex decision-making. The approach is summarized in Figure 1.
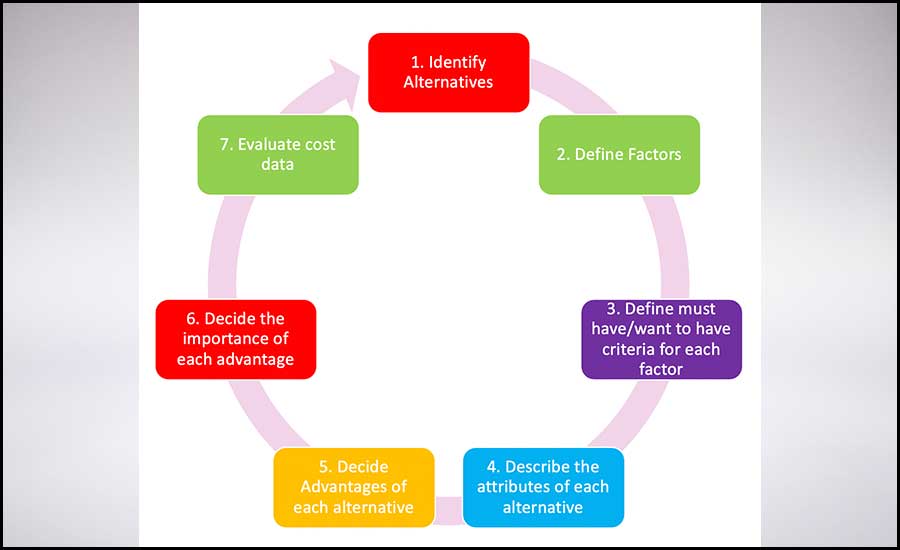
In this paper, a hypothetical case study is developed using CBA as it applies to pandemic-ready HVAC alternatives. The case study involves the selection of systems for an office building. The first step in this case study is the selection of the alternatives to be considered. ASHRAE’s position statement is a good starting point: “Airborne transmission of SARS-CoV-2 is significant and should be controlled. Changes to building operations, including the operation of HVAC systems, can reduce airborne exposures. Ventilation and filtration provided by HVAC systems can reduce the airborne concentration of SARS-CoV-2 and thus the risk of transmission through the air.”
The design recommendations of the ASHRAE Epidemic Task Force provide a basis for considering alternatives for pandemic-ready measures. The following is a summary of those suggestions:
- Provide and maintain, at the very least, the required minimum outdoor airflow rates for ventilation as specified by applicable codes and standards.
- Use a combination of filters and air cleaners that achieve MERV 13 or better levels of performance for air recirculated by HVAC systems.
- Select-control options, including stand-alone filters and air cleaners, that provide desired exposure reduction while minimizing associated energy penalties.
Each of these recommendations has many possible technical approaches but these may conflict with other project requirements, such as energy use. CBA provides a sound method of analyzing these options. Based on the ASHRAE recommendations, the following alternatives are reviewed:
- Increasing outside air above local code requirements to achieve the lowest probability of infection (enhanced outside air).
- Increasing filtration from MERV 8 to MERV 13 at the air handler to achieve the lowest probability of infection (enhanced filtration).
- Supplemental bipolar ionization (BPI) for a typical air-handling system using MERV-8 filters.
Additive air purifying at the zone level of the office was considered, but this can be included in each of the alternatives, so it was not a mutually exclusive option.
Next, we consider the factors we wish to evaluate for each alternative. Factors are elements or components of a decision that contain the criteria, attributes, and advantages required for making it. This exercise reviews the following factors:
1. Pandemic-readiness;
2. Safety;
3. Maintainability; and
4. Energy.
Many other factors, such as sound, controllability, and flexibility, could be considered. However, a key principle of CBA is identifying factors that reveal important differences between the attributes of the alternatives. The four factors and the alternatives are used in the CBA tabular method as seen in Table 1. For each factor, the desirable criterion is defined, as shown in Table 1. A criterion is a standard in which a judgment is based. In CBA, they are classified as a “must-have” or “want to have” criterion. If an alternative does not comply with a “must-have” criterion, it must be discarded.

The decision-making steps (Nos. 4 thru 7 in Figure 1) come next. Step four describes the attributes for each alternative with respect to the factors listed. Attributes are defined as characteristics of an alternative. The advantage of an alternative is the beneficial difference between the attributes of two alternatives (one of which is the least preferred).
Determining the Importance of the Advantages
Suhr emphasizes the importance of anchoring decisions based on relevant facts. Critical to this is deciding the importance of the advantage between the alternates:
- Apply positive values to all advantages;
- Weigh the advantages on the same scale; and
- Decide (don’t calculate) the importance of an advantage.
The importance is based on the following:
- The importance and circumstance of the decision;
- The needs and preferences of all of the stakeholders;
- The magnitudes of the advantages; and
- The magnitudes of the associated attributes.
These considerations are explored in the case study. The costs of the alternatives are not considered until the end of the process — after the advantages have been evaluated. In this paper, the costs are not evaluated. The important distinction of using CBA from other methods is that CBA focuses only on the comparison between advantages of alternatives. It does not review disadvantages of alternatives because they can always be framed as an advantage of another alternative, nor introduce biases by preemptively weighting factors in the decision-making process. This eliminates double-counting errors and provides a sound platform for choosing among several alternatives. Under the factor of pandemic-readiness, the attributes are (Table 2) listed for each alternative.
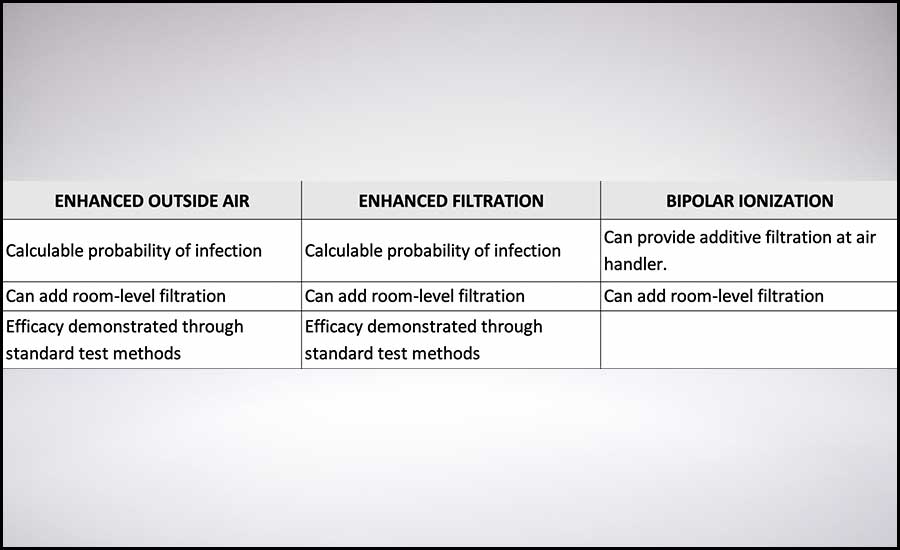
Because the criteria for this factor is “less probability of infection is better,” the probability of infection of each alternative must be quantified to make a sound decision. As a result, the Wells-Riley equation, which quantifies the probability of infection, plays a crucial role in choosing the best option.

Where: P = probability of infection I = number of infected people q = production rate per infector (quanta/hour) p = pulmonary ventilation (m3/hour) t = time (hours) Q = ventilation rate of “germ free” air (m3/min) The variable affected by outside air and filtration is Q. Outside air is assumed to be “germ-free.” As shown by Elovitz, 30 cfm of MERV-13 filtered, recirculated air is equivalent to 20 cfm of “germ-free” air supply. This relationship is used under the energy factor, but it shows that outside air and filtration can achieve similar quantifiable probabilities of infection. For example, a recent study of an outbreak in a restaurant in Guangzhou, China, estimates a “germ-free” ventilation airflow of 82 cfm would have been required to protect the restaurant’s patrons. This would be the equivalent of 123 cfm of MERV-13 filtered air. Thus, both options provide the equivalent probability of infection against infectious disease but with different total airflow rates to the space. As a result, the advantages of the options of enhanced outside airflow and filtration are graded as equal. However, in the case of BPI, with no independent, scientific studies demonstrating its effectiveness in lowering probability, it presents the least preferred attribute, and, therefore, it has no advantage in this factor.
For the energy factor, the criterion “less energy use is better” reigns true. The analysis of the alternatives reviews the energy consumption required to achieve equivalent probabilities among alternatives.
For Outside Air the energy required is:

We have ignored the energy associated with latent heat removal at the coil in equation 1. Assuming a variable air volume (VAV) system and an ASHRAE 90.1-compliant fan with a Fan Energy Index (FEI) of 95 (an assumed fan efficiency of 62%), the relation between energy consumption and enhanced filtration is:
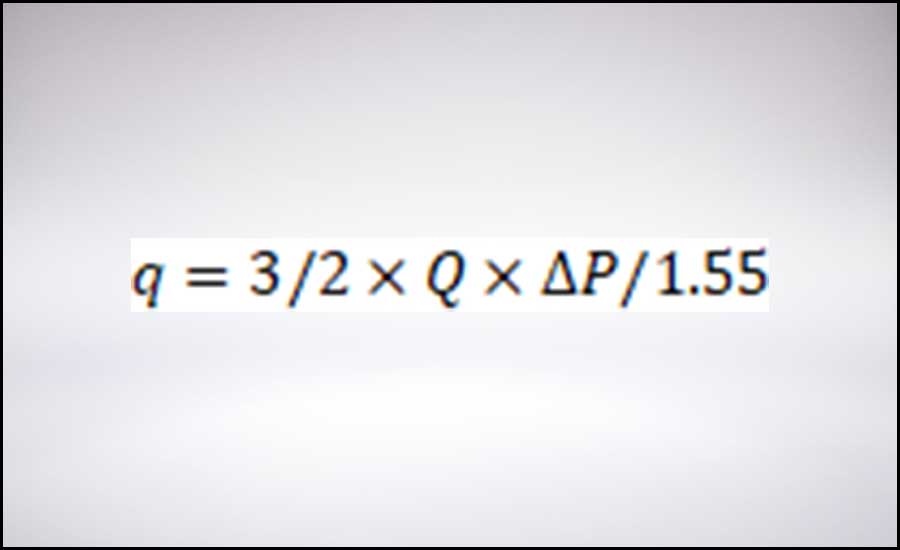

Finally, for BPI, the relation between energy consumption and airflow is inconsistent between manufacturers. After review of several manufacturers’ literature, this paper uses a power consumption of 50 W/1,000 cfm. Assuming that BPI can achieve the same level of probability of infection treating a quantity of recirculated air equivalent to outside air (an unsubstantiated assumption), the energy used per cfm is:


Where:
q = energy Btu/hr
Q = outside airflow (cfm)
∆T = temperature rise of air (°F)
∆P = total pressure drop across the filter (in. w.g.)
Assuming that adding MERV-13 filters will add a 0.25 in w.g. pressure drop to the system, equations 2 and 4 are equated:


To achieve the same probability of infection with an energy consumption increase equivalent to enhanced filtration, the outside air temperature can only change by .01°F under the enhanced outside air alternative, indicating that in any climate where outside air must be conditioned, this alternative will spend considerably more energy as opposed to enhanced filtration to lower the probability of infection.
Next, enhanced ventilation is evaluated against BPI for energy use. Comparing equations 3 and 5 provides:
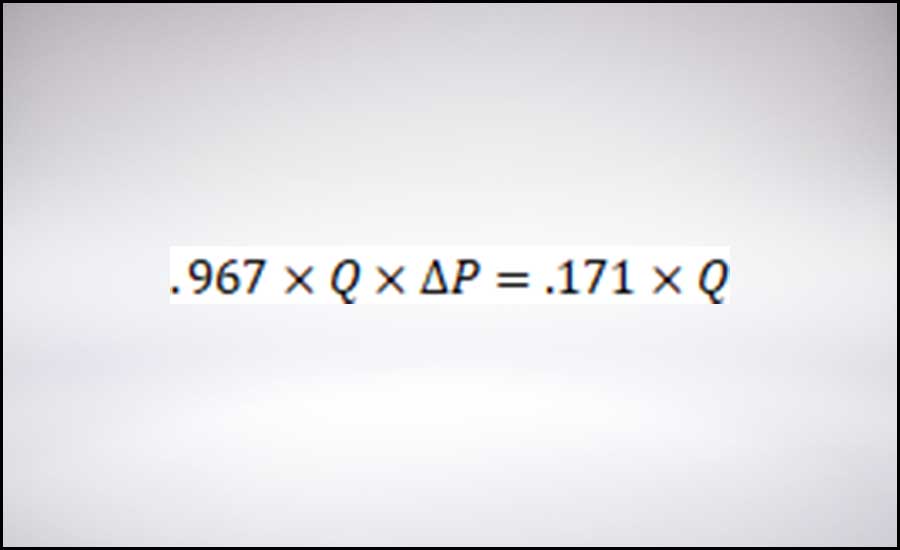

Assuming a 0.25 in. w.g. drop for enhanced ventilation, the energy consumption for BPI is slightly less than enhanced filtration. However, the uncertainty in the assumption that BPI is providing equivalent probability of infection at this energy consumption reduces the importance as well as the magnitude of the difference of the advantage as seen in Table 3.
Similar exercises for safety and maintainability factors are performed. Under safety, the fact that outside air and filtration are devoid of possible byproducts provides an advantage, while BPI is perceived by the team to have the fewest additional requirements with respect to maintainability and, in fact, benefits from both advantages offered by the other alternatives. Note that none of the alternatives is considered a significantly risky option in terms of safety.
The paramount advantage is determined by the team to be “pandemic-ready.” This is the “need.” The remaining advantages are “wants.” The advantages are given scores based on their importance and the results are shown in Figure 4. As such, the alternative with the greatest pandemic-ready advantage scores 100 points. The underlined attributes are the least preferred attributes for the factor. The advantage and importance are listed on the line below the attributes. Note that none of the alternatives are considered a significant risk in terms of safety. Thus, the scoring for safety is less than the paramount advantage of pandemic readiness.

As shown in Table 3, enhanced filtration is the preferred method. Because the team placed an emphasis on quantifiable reduction in infection probability, BPI suffered, since current scientific data does not allow this evaluation. Nevertheless, CBA does not eliminate subjective evaluation of the alternatives and, instead, focuses the decision-making on relevant facts as discovered by the stakeholders. Thus, the results may be different for different teams or in different contexts. The final step is considering the costs for each alternative as they relate to the advantages. In many cases, the advantages will be deemed to outweigh costs of an alternative.
As seen in this example of evaluating systems in relation to pandemic readiness, CBA provides a framework to make sound decisions. On a broader level, it can be applied any time the engineer is confronted with multiple solutions to a problem, which is true of every project. This is the bigger lesson of this paper — making sound decisions requires effective systems for doing so. In an industry where projects begin with choosing among alternatives, why wouldn’t the use of such a system be more pervasive?
References
- Varley, J. (2020). Streamline the Modeling Process by Exporting Data Within BIM, Engineered Systems, 37 (12).
- Arroyo, P. (2014). Exploring decision-making methods for sustainable design in commercial buildings. University of California, Berkeley.
- Suhr, J. (1999). The Choosing By Advantages Decision-Making System. Quorum, Westport, CT.
- Arroyo, Paz (2015). Step By Step Guide To Applying Choosing By Advantages Tabular Method, https://paramountdecisions.com/blog/step-by-step-guide-to-applying-choosing-by-advantages
- ASHRAE, COVID-19: Resources Available to Address Concerns (ashrae.org)
- ASHRAE Epidemic Task Force (2021). Core Recommendations for Reducing Airborne Infectious Aerosol Exposure.
- Riley, E C, Murphy, G (1978). Airbourne spread of Measles in a Suburban Elementary School, Am. J. of Epidemiology, 107 (5).
- Elovitz, K. and Elovitz, G. (2020). Assessing the Role of HVAC Systems in Fighting COVID-19, HPAC Engineering, 92 (9).
- Li Y, Qian H, Hang J, et al. (2021). Probable Airborne Transmission of SARS_CoV-2 in a Poorly Ventilated Restaurant, Building and Environment, (196).
- ASHRAE. Filtration / Disinfection (ashrae.org)
- American Society of Heating, Refrigerating, and Air-Conditioning Engineers. (2019) Standard 90.1 – Energy Standard for Buildings Except Low-Rise Residential Buildings. Atlanta: ASHRAE.
- Zeng Y, Manwatkar P, et al. (2021). Evaluating A Commercially Available In-Duct Bipolar Ionization Device for Pollutant Removal and Potential Byproduct Formation, (195).