

FIGURE 1. Two percent ground acceleration map. (Courtesy, www.usgs.gov.)
Professional architects and engineers must determine reasonable anticipation and the associated cost implications of designing buildings to worst-case scenarios. Building codes must address these issues. Building insurance companies must analyze the risk of insuring buildings subjected to these forces. Each decision carries a level of risk and liability that someone must accept.
A seemingly simple roof curb for a duct termination or fan can create liability for an engineer, contractor, and owner if there is no regard to the code requirements for seismic and wind forces. This article discusses some information that will be beneficial to consider, although it is not an exhaustive discussion of the material and research available.
Seismic Hazard Maps
In order to understand the statistical significance of why building codes are as they are, a good resource is the USGS. An interesting website at http://earthquake.usgs.gov/earthquakes/recenteqsus/ provides a map of earthquakes in the U.S. in the last seven days. The website also has other maps showing such statistics as deadly earthquakes, earthquake density, historic earthquakes, last earthquakes in each state, etc.Another good source for understanding more about earthquakes is NEHRP (www.nehrp.gov), which is maintained by NIST on behalf of FEMA, NSF, and USGS.
It is interesting to note that while the frequency of earthquakes is not as great there, the potential magnitude of forces is greater in the central U.S. than in the western part of the country. This lack of frequency in the central U.S. has often led small local municipalities to overlook the seriousness of the potential danger of another major earthquake.
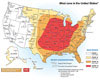
FIGURE 2. Wind zones in the United States. (Courtesy, http://www.fema.gov/plan/prevent/saferoom/tsfs02_wind_zones.shtm.)
Structural Actions
The structural engineer on a project, in coordination with the mechanical engineer, must ensure that the load path of all equipment mounted on and in the building is transferred to the structure such that these components will not cause damage to the building if they were forced off of their mountings. (ASCE 7-1.3.5 Counteracting Structural Actions.)1.3.5 Counteracting Structural Actions. All structural members and systems, and all components and cladding in a building or other structure, shall be designed to resist forces due to earthquake and wind, with consideration of overturning, sliding, and uplift, and continuous load paths shall be provided for transmitting these forces to the foundation. Where sliding is used to isolate the elements, the effects of friction between sliding elements shall be included as a force. Where all or a portion of the resistance to these forces is provided by dead load, the dead load shall be taken as the minimum dead load likely to be in place during the event causing the considered forces. Consideration shall be given to the effects of vertical and horizontal deflections resulting from such forces. (Ref.: IBC 2009)
Equipment Certificate Of Compliance
The IBC directly assigns a duty to the registered design professional to take specific action with respect to ensuring that the manufacturer of a product has appropriate compliance to the code requirements. This is a discussion between the owner and the engineer, and the owner and his building insurance provider, who may not cover damage caused by products not in conformance with this code requirement. Suppliers and contractors who ignore this part of the code must realize there is also an added risk and liability to their businesses. (IBC 2009-1708.4 Seismic Certification of Nonstructural Components).1708.4 Seismic certification of nonstructural components. The registered design professional shall state the applicable seismic certification requirements for nonstructural components and designated seismic systems on the construction documents. 1. The manufacturer of each designated seismic system component subject to the provisions of ASCE 7 Section 13.2.2 shall test or analyze the component and its mounting system or anchorage and submit a certificate of compliance for review and acceptance by the registered design professional responsible for the design of the designated seismic system and for approval by the building official. Certification shall be based on an actual test on a shake table, by three-dimensional shock tests, by an analytical method using dynamic characteristics and forces, by the use of experience data (i.e., historical data demonstrating acceptable seismic performance) or by more rigorous analysis providing for equivalent safety. 2. Manufacturer’s certification of compliance for the general design requirements of ASCE 7 Section 13.2.1 shall be based on analysis, testing or experience data. (Ref. IBC 2009)
The IBC code is a building performance code. If the MEP systems are designed to the same seismic design force as the building itself, then those MEP systems will continue to operate after a seismic event. Equipment manufacturers need to guarantee on-line performance through independent testing and analysis as outlined in the code.
This requirement for manufacturers, and consequently their equipment dealers, is a change from all previous building codes. Now when a fully functional system is required by the code, equipment manufacturers and dealers are held liable for the equipment performance after seismic or wind events. Engineers who specify equipment must take this into account in their product specifications and submittal review process.
According to the IBC, when an MEP engineer deems a product as a designated seismic system component, then the manufacturer must supply a certificate of compliance stating that the equipment will meet the applicable seismic design criteria for that project. The basis for this compliance can be done by shake-table testing or other analyses such as finite element modeling. Historical data may sometimes also be accepted; however, many states have removed this from their adopted state code.
The equipment manufacturer must also supply a label to the equipment that contains sufficient information for the inspector to determine that the installed product is the same as that which was approved during plan review. The special inspector acts on behalf of the building owner or MEP engineer and verifies that the labeling of the equipment and anchorage or mounting conforms to the previously supplied manufacturer certificate of compliance. Additionally, the AHJ will look for product labeling and certificates of compliance.

FIGURE 3. (Top left) Example of AMCA 511 wind drive rain and hurricane louvers. (Photo courtesy of Ruskin Corp.) (Top right) Example of a horizontal blade AMCA 511 wind drive rain louver. (Photo courtesy of Ruskin Corp.) (Bottom left) Example of color-coded seismic cables. (Photo courtesy of Loos & Co.) (Bottom right) Examples of seismic mounts. (Photo courtesy of The VMC Group.)
Special Inspections - Engineer Responsibility By Code
The IBC addresses an aspect of seismic design and installation that is very likely overlooked by many professional engineers simply because it may not be enforced by the AHJ. However, the code puts the liability squarely on the “registered design professional in responsible charge” to employ an inspector during construction. Just because the AHJ doesn’t enforce this does not relieve the engineer from this code requirement. This should be discussed in the owner/engineer agreement and resolved prior to doing engineering work for any client. The owner in turn would be wise to discuss this with their building insurance carrier to determine if omitting this from a contract with an engineer would void any aspect of their building insurance coverage.1704.1 General. Where application is made for construction as described in this section, the owner or the registered design professional in responsible charge acting as the owner’s agent shall employ one or more approved agencies to perform inspections during construction on the types of work listed under Section 1704. These inspections are in addition to the inspections identified in Section 110. (Ref: IBC 2009)
Importance Factor
It is essential for the HVAC engineer to understand that the component importance factor (Ip) is required to be established and clearly documented in the contract document for every HVAC system. The occupancy categories are the starting point to establishing the Ip, and then the system within the building determines if the Ip should be a 1.5 or a 1.0, the only two values for this factor (ASCE 7-13.1.3 Component Ip).13.1.3 Component Importance Factor. All components shall be assigned a component importance factor as indicated in this section. The component importance factor, Ip, shall be taken as 1.5 if any of the following conditions apply: 1. The component is required to function for life-safety purposes after an earthquake, including fire protection sprinkler systems. 2. The component contains hazardous materials. 3. The component is in or attached to an Occupancy Category IV structure and it is needed for continued operation of the facility or its failure could impair the continued operation of the facility. All other components shall be assigned a component importance factor, Ip, equal to 1.0.” (Ref. ASCE 7-05)
In general, all MEP hospital components (e.g., heating and air conditioning systems, generator sets, transfer switches) need to be certified to this IBC guideline. Since hospitals are classified as an “essential facility” and most, if not all, of its components have an Ip of 1.5, compliance becomes a requirement for most, if not all, of its operational systems.
Consequential Damage - Crucial Coordination
Just how important is coordination of HVAC systems when designing and installing? Very important. If an HVAC system that is assigned an Ip of 1.0 is installed above a system that is assigned an Ip of 1.5, and the 1.0 system could break loose in a seismic event and take down the lower system, then the upper system would also need to be installed with bracing as if it were a system with an Ip of 1.5 to prevent “consequential damage” (ASCE7-13.2.3 Consequential Damage).13.2.3 Consequential Damage. The functional and physical interrelationship of components, their supports, and their effect on each other shall be considered so that the failure of an essential or nonessential architectural, mechanical, or electrical component shall not cause the failure of an essential architectural, mechanical, or electrical component. (Ref.: IBC 2009)

FIGURE 4. An example of an inadequately fastened gooseneck duct termination, which can be problematic in a wind and rain storm. Water can enter the building through the duct system, causing extensive damage.
Wind - Don't Blow It Off
Seismic forces aren’t applicable to every part of the country, but wind forces are. The code is very clear about the responsibility to take wind forces into account in the building and building system design. Architects and structural engineers may be more in tune with this than some mechanical engineers; however, the HVAC system components can be a very likely risk if not properly supported and designed.The Federal Emergency Management Agency (FEMA) has developed Wind Zone maps for the U.S. These maps show the three wind gust zones for the various areas of the country. These wind gusts are the basis of consideration for the development of the building codes.
A seemingly simple installation of a “gooseneck” duct termination can be problematic in a wind and rain storm. It isn’t just the damage to the roof and equipment that is of concern; it also becomes a more serious concern when water can enter a duct system and distribute in a building causing extensive water damage throughout it. Figure 4 shows an example of how an inadequately fastened HVAC duct can be blown over, subsequently subjecting the duct system to rain that can cause a large about of water damage in a building and subject the building to potential mold problems even when the duct is fixed. This is a liability that no engineer, architect, contractor, or building owner wants to take unnecessarily by not complying with building codes.
One detail and specification coordination item that should be reviewed on every project is the structural integrity of all rooftop HVAC equipment, ducts, and piping on a roof. This is an analysis that should be part of the contract documents so that the fastening integrity of these items is not left up to the discretion of the contractor without proper engineering analysis to determine the quantity, quality, and size of all fasteners.
Care should be taken to ensure that the curb and supports are also of sufficient material construction to withstand the forces at the fastener penetrations. This is an analysis that is likely going to require a structural engineer. Most fan manufacturers do not give any recommendations on the number of sized or spacing of fasteners to hold a fan to a curb, so it is not sufficient to rely on “manufacturer’s recommendations” without a clear communication of the forces that the connection is to withstand in accordance with the code requirements.
Louvers
A growing number of mechanical engineers are taking charge of the louver specifications since these are air-handling type products that require specification of both pressure loss and water penetration rates as part of the HVAC system. AMCA Publication 511 has a section for “Wind-Driven Rain Test” which tests and gives louvers a rating for both effectiveness ratio of water blown through the louver and a Class rating for specifications at both 29 mph wind velocity with 3 in./hr and also 50 mph wind with 8 in./hr of rain.Keeping water out of buildings has become a high priority in building designs in recent years, and this holds true for mechanical systems where water can enter OA plenums and accumulate if proper water management is not designed into the system. Water blown through louvers can also saturate air filters, which can then fall out of place. Specifications of louvers also must take into account the structural stability of the louver, using the same criteria by which the building wind forces are determined by code requirements. There are also hurricane-rated louvers, but they are not within the scope of this article.
HVAC Equipment And Systems Considerations
Just how much does an HVAC engineer need to know about seismic and wind issues to design and specify equipment and supports to comply current industry standards, codes, and standard of care? In general, most mechanical engineers will likely utilize another engineer who specializes in seismic design services. An engineer who engages in seismic design without the knowledge and competence to do this type of engineering would likely be using his seal in a manner which would not be in conformance with the state statute in which he is practicing.The forces exerted on buildings during earthquakes cannot be stopped; however, they can be dealt with in a manner that reduces risk and liability and keeps damage to a minimum. Seismic bracing reacts to these forces keeping the HVAC services in place to ensure the building occupants remain safe.
A seismic mount is more than an isolator. An isolator is a product designed to “isolate” the vibration of a piece of rotating equipment or pipe or duct connected to a piece of rotating equipment from the building structure for the purpose of eliminating or minimizing the vibration transmitted to the structure. A seismic mount is a product designed to prevent the piece of equipment from being forced out of place during a seismic event, so that the piece of equipment won’t cause damage by being moved out of place (or in some cases, ensure that the piece of equipment will stay functional during and after the seismic event).
The seismic isolator must restrain the equipment from both lateral and overturning forces placed upon it as the seismic wave shakes the building structure and transmits these forces onto the equipment supports. A seismic restraint can (and often does) function as an isolator also.
Points To Ponder
Every HVAC engineer must take a close look at design practices, contract document formats, errors and omissions insurance coverage, client agreements, design coordination process, and submittal review process to ensure that the HVAC products and systems are in compliance with standards and codes in order to minimize their risk and liability. Although there may be a tendency to just do what the AHJ enforces, this does not relieve the professional engineer from his professional obligations.To minimize risk and liability, equipment manufacturers, suppliers, design professionals, and installing contractors need to clearly understand their roles and responsibilities as defined in chapters 16 and 17 of the IBC code. In recent years, the insurance industry has put the mechanical and electrical equipment industry on notice. Studies have been done post-Northridge and Hurricane Katrina, proving that buildings designed to the most current building codes have a higher survival rate than those which were not.
The industry concluded that proper design and installation does reduce insurance losses and that failure to properly design and install equipment will lead to lawsuits to recover part of their losses. Policy writers are expected to set premiums based on a building owner’s adherence to the code. Insurance claims on MEP systems will be reduced if equipment has been designed to the same seismic and wind load criteria as the building. The cost to replace or repair the MEP systems within buildings is likely the most expensive cost to insurers after a seismic event.
Will the insurance industry be willing to pay out claims for equipment that was not in compliance with the building code requirements, or if related inspections (IBC 2009-1704.1) were not carried out during construction as proscribed? This is yet to be seen, but who is willing to continue to take the risk, and for what reason?ES
Sidebar: Working With Newton
(Since this isn’t a frequent feature topic, we asked a couple of manufacturers in this area to weigh in with sidebars on seismic issue as well. Here’s the first, from Metraflex.)Buildings aren’t dynamic. Ideally, they are supported by solid, non-accelerating ground. During a seismic event, this is not the case. The earth supporting the structure is accelerating, sometimes violently. Building foundations are also accelerating, and our once static structure is suddenly dynamic and very much under the control of Newton’s Second Law (F=ma).
A seismic event not only moves buildings, but the stuff inside the buildings also accelerates. This includes the electrical, plumbing, fire and HVAC systems. The pipe, sheet metal and equipment that were peacefully hanging from the ceiling slab are now subject to dynamic forces that can be several times the force of gravity. Critical building systems and their associated supports must be able to withstand these dynamic forces.
Design of this equipment and supports is addressed in Section 1613 of the 2009 IBC. Specifically, the IBC refers to ASCE 7 (Minimum Design Loads for Building and Other Structures) for the minimum design requirements of nonstructural components permanently attached to structures and their supports and attachments.
For example, structural engineers routinely design buildings with seismic joints, which allow sections of buildings to move several inches with respect to each other during seismic events. It is also common to have pipes cross these joints. If this movement is not addressed by the MEP/FP engineering team, pipes and supports crossing a seismic joint can be subject to damaging displacements in addition to the accelerations imposed on the system.
Pipe expansion joint manufacturers have devices to accommodate this movement. Some examples include universal tied bellows assemblies, hose and braid loops, ball joint assemblies and grooved coupling assemblies. The specifying engineer must coordinate with the structural engineer to determine the amount of building movement, and research the available devices to select the best device for the application.
Structural engineers are getting better at designing buildings to withstand major earthquakes. It is now up to the MEP/FP community to be familiar with the seismic codes and design critical building systems that remain intact and functioning after “the big one.”
Sidebar: Just Enough Swing
(Here, Victaulic provides some additional input, in the second manufacturer sidebar we requested to accompany this fairly rare feature on seismic concerns.)In piping, there are a number of off-the-shelf grooved end products designed to accommodate seismic movement. Dave Hudson, a senior engineer for Victaulic, answers some questions about piping strategies, taking into consideration building sway, inter-story drift, and building seismic joints.
What are the best ways to pipe a building to accommodate seismic activity?
The main approach to piping a building to accommodate seismic activity is to go with a rigid piping system to minimize overall piping movement. Too much swing could affect other pipe and equipment despite being within the capability of the grooved coupling. Typically, more than 95 percent of the grooved couplings used in a system will be rigid, with the remainder as flexible couplings that allow linear, angular, and rotational movement in areas as needed.
With seismic joints, how can contractors accommodate differential movement to prevent damage?
Seismic joints allow building sections to move differentially during an earthquake. With grooved systems, contractors can prevent damage by using a seismic swing joint or multiple flexible couplings using piping geometry. The exact method is determined by the size of the pipe and the amount and type of movement to be accommodated.
Where are typical locations for piping seismic joints?
Typical locations for piping seismic joints would be at building seismic joints, between floors, to accommodate inter-story drift, and also where piping enters base isolated buildings.
How are swing joints mounted?
Swing joints accommodate a considerable amount of movement. They are usually hung with spring hangers or cable supports. Also, the piping on either side of the swing joint must be anchored, so that the movements are isolated to the swing joint.